Abstract
INTRODUCTION
Serum S100β levels are mostly used for predicting outcomes of large-vessel stroke. Its application to mixed subtypes of acute ischaemic stroke (AIS) has been limited.
METHODS
Patients with mixed subtypes of AIS who were aged over 18 years and presented within 24 hours of stroke onset were consecutively enrolled. Serum S100β levels at presentation (S100βb) and 72 hours (S100β72hrs), and corresponding National Institutes of Health Stroke Scale (NIHSSb and NIHSS72hrs, respectively) scores were assessed. Stroke outcomes were evaluated using the modified Rankin Scale (mRs) at 30 days (mRs30) and 90 days (mRs90). Correlations between S100βb and S100β72hrs, as well as differences between the two (∆S100β) and the corresponding NIHSS, mRs30 and mRs90 scores, were evaluated (p < 0.05).
RESULTS
35 patients were eligible for analysis. On univariate analysis, stroke outcomes had a significant association with S100βb, S100β72hrs, NIHSSb, NIHSS72hrs and ∆S100β. Both S100βb and S100β72hrs correlated with corresponding NIHSS values (ρb = 0.51, p < 0.001; ρ72hrs = 0.74, p < 0.001), mRs30 (ρb = 0.58, p < 0.001; ρ72hrs = 0.72, p < 0.001) and mRs90 (ρb = 0.51, p = 0.002; ρ72hrs = 0.68, p < 0.001). Correlations existed between ∆S100β and mRs30 (ρ = 0.74, p < 0.001) and mRs90 (ρ = 0.71, p < 0.001). Practical cut-off points for unfavourable outcomes (mRs 3–6) were S100β72hrs > 0.288 µg/L (sensitivity 92.3%, specificity 86.4%) and ∆S100β > 0.125 µg/L (sensitivity 100%, specificity 81.8%).
CONCLUSION
High serum S100β is associated with unfavourable outcomes for mixed subtype AIS. Cut-off values of S100β72hrs and ∆S100β were optimal for predicting unfavourable stroke outcomes.
INTRODUCTION
Various neural-specific biomarkers are released into the serum and cerebrospinal fluid during neural tissue injuries. Both direct and indirect cerebral damage (e.g. cerebral trauma, cardiopulmonary arrest, postoperative neurovascular complications and acute ischaemic stroke [AIS]) lead to the release of these biomarkers.(1-5) Thus, assessment of the presence and magnitude of such biomarkers during neurovascular insults may facilitate not only the diagnosis but also the prediction of the complications and final outcomes of the neural injury.(1,2,6) A recent study found that each biomarker has a specific cellular origin within the cerebral tissue (e.g. neuron-specific enolase [NSE] is released from neurons; serum S100β is released from astrocytes and Schwann cells; Tau protein is from neurons; metalloproteinase-9 is released from vascular endothelium; and ubiquitin C terminal hydroxylase-L1 is released from neurons).(3) Therefore, several biomarkers associated with specific cerebral cellular damage have been under extensive investigation to identify novel biomarkers that could serve these purposes.(7) Recently, among many stroke-related biomarkers that were widely evaluated for their suitability for clinical deployment, S100β and NSE were the two most broadly attributed in many clinical studies for their clinical usefulness.(2,3,6,8-12) Because of the greater clinically correlated response of S100β and absence of interference by the body’s clearance systems, there has been much interest in proving its clinical applicability.(3)
The S100β protein is an acidic, calcium-binding, neural-specific biomarker. It is a ~21-kDa protein with a homodimeric structure (double helix loop) that is encoded on the long arm of chromosome 21 (21q22.3).(2,13) As S100β has been used as a target of immunologically specific staining techniques to demonstrate astrocytes and melanocytes, it is a potentially promising biomarker for damage to astroglial cells as well as melanocyte proliferation.(3,7) Furthermore, a study based on experimental induction of endothelial injury has suggested that S100β could be a biomarker for blood-brain barrier disruption.(14)
Several studies have reported a significant elevation of serial S100β levels following AIS, in particular among patients with large-vessel or cortical brain infarction. The elevation of S100β, both initially and subsequently at the appropriate time points, significantly correlated with not only the extent of cerebral infarction, as reflected by stroke severity, but also the associated complications and neurological outcomes.(8-12,15,16)
Earlier studies have reported that S100β was first detected in the serum of patients with AIS at 6–12 hours and reached peak levels at 72 hours after the onset of AIS.(12,17,18) It followed first-order kinetics, with a biological half-life of 25.3 ± 5.1 minutes, without effect from a moderately decreased glomerular filtration rate.(5) Nevertheless, due to the delayed kinetics for the first detection in serum (6–12 hours), S100β is not an ideal biomarker for AIS diagnosis.(12,18)
To the best of our knowledge, there has been limited evaluation of the clinical usefulness of S100β in mixed cortical (or large-vessel occlusive stroke [LVS]) and subcortical (or small-vessel occlusive stroke [SVS]) AIS. Therefore, in the present study, we aimed to: (a) demonstrate the significance of the correlations between the initial and subsequent (at 72 hours after AIS onset) S100β levels with the severity and outcomes of patients with mixed subtype AIS; and (b) determine the optimal cut-off points of S100β levels at each time point for predicting unfavourable outcomes in patients with mixed subtype AIS. We intended to prove the clinical applicability of S100β for predicting the outcomes of patients with mixed cortical and subcortical ischaemic strokes.
METHODS
We prospectively enrolled all patients aged over 18 years who presented to Songklanagarind Hospital, Faculty of Medicine, Prince of Songkla University, Songkhla, Thailand, from January 2013 to September 2013 with newly diagnosed AIS within 24 hours of the onset of symptoms. The diagnosis of AIS was based on the patient’s clinical presentation and confirmed by either computed tomography or magnetic resonance imaging of the brain. Patients with a previous history of neurological illness, existing neurological disorder or residual neurological disability from any cause (e.g. stroke, cerebral neoplasm, intracranial infection or traumatic cerebral injury) were excluded. To achieve statistically significant power, a sample size of 36 was necessary based on the equation:
where proportions of positive outcomes among the exposed group (p1) = 0.73 and proportions of positives outcome among the non-exposed group (p2) = 0.17, a = 0.05, power = 0.8, and r = 1.
Patients’ demographic data and cardiovascular risk factors were collected during stroke risk screening. Presenting stroke severity was graded using the National Institutes of Health Stroke Scale score (NIHSS) immediately upon the patient’s arrival at our centre, as the baseline severity scale (NIHSSb). An emergency cranial imaging study, in most cases computed tomography of the brain, was performed to confirm the diagnosis and classify the subtype of AIS, in combination with the clinical stroke syndrome. All brain images were subsequently reviewed and confirmed by a clinically blinded neuroradiologist. The first blood sample for the baseline assay of S100β (S100βb) was collected prior to starting treatment.
The primary AIS treatment consisted of one or a combination of the following: intravenous thrombolysis, endovascular thrombectomy and antithrombotic agents. The second S100β assay was performed at 72 hours after the onset of stroke symptoms (S100β72hrs), together with a re-evaluation of stroke severity using NIHSS (NIHSS72hrs). Stroke-related complications (e.g. haemorrhagic transformation and malignant cerebral oedema necessitating decompressive craniectomy) were monitored. Stroke outcomes were assessed using the modified Rankin Scale (mRs) at 30 days (mRs30) and 90 days (mRs90) after AIS onset. mRs ≤ 2 was considered as favourable, while mRs 3–6 was unfavourable.
Measurement of serum S100β levels was performed using a commercial kit (Elecsys S100; Roche Diagnostics, Indianapolis, IN, USA) that was composed of a two-site monoclonal S100-specific antibody to form a sandwich complex on a fully automated system (Elecsys 2010, Modular Analytics E170; Roche Diagnostics-Hitachi High-Technologies Corporation, Tokyo, Japan). The range of measurement was 0.015–30.0 µg/L. Values were evaluated within an intra-assay coefficient of variation in the range of 1.28%–2.32%.
Descriptive statistics were shown as number and percentage, mean ± standard deviation or median (interquartile range [IQR]). Fisher’s exact test and chi-square test were used to analyse categorical variables, whereas Student’s t-test and Wilcoxon rank-sum test were used to analyse continuous variables for statistical significance. A p-value < 0.05 was considered to be statistically significant. Continuous variables were assessed using the Shapiro-Wilk test for normality. Correlations between S100βb and S100β72hrs and the corresponding NIHSSb and NIHSS72hrs values as well as correlations between each S100β level and the difference of the two S100β measurements (∆S100β) with mRs30 and mRs90 were evaluated using Spearman’s correlation. The optimal cut-off points of S100βb, S100β72hrs and ∆S100β levels to predict final patient outcomes and mortality were obtained using receiver operating characteristic (ROC) curves. The sensitivity, specificity, positive predictive value and negative predictive value of each S100β and ∆S100β cut-off point obtained were also calculated.
The complete study protocol was reviewed and approved by the ethics committee of the Faculty of Medicine, Prince of Songkla University, Songkhla, Thailand. We strictly followed the regulations of the 1964 Declaration of Helsinki and its later amendments while performing the study. All identifiable personal information of enrolled patients was made completely anonymous.
RESULTS
In total, 35 out of 44 enrolled patients with AIS were eligible for final analysis after excluding six patients who met the exclusion criteria and three patients who had incomplete follow-up. There were 26 men and nine women with an overall mean age of 65.3 ± 10.7 (range 43–81) years (
Smoking, hypertension and hyperlipidaemia were sequentially the three most common cardiovascular risk factors among our patients. The total number of SVSs was 17 (48.6%), involving 15 SVSs and two other undetermined subtypes that were finally classified as SVSs. The median NIHSSb was 10.0 (IQR 6.0–19.0), while the median NIHSS72hrs was 6.0 (IQR 2.5–16.0). The S100βb assessments were available at a mean time of 10.6 ± 7.3 (range 2.0–24.0) hours after stroke onset, while the S100β72hrs assessments were performed at 72 hours after stroke onset.
For primary stroke treatment, 21 patients received conventional therapy in the form of antiplatelet or anticoagulant therapy, while ten patients received intravenous thrombolysis and one patient underwent endovascular thrombectomy. Primary stroke treatment was withheld for three patients – two patients had haemorrhagic transformation and one patient had massive cerebral oedema initially. The final stroke outcomes evaluated using mRs30 and mRs90, which were categorised as favourable (mRs ≤ 2) or unfavourable (mRs 3–6), were identical for the categorical outcomes (favourable or unfavourable) at both time points for each patient. Five patients had complications of haemorrhagic transformation, and two patients developed malignant brain oedema for which decompression craniotomy was indicated. Eventually, 22 patients had a favourable outcome, while 13 patients had an unfavourable outcome. Six of the patients died of direct stroke-related severity and complications.
S100βb, S100β72hrs, ∆S100β, NIHSSb and NIHSS72hrs were significantly associated with categorical stroke outcomes on univariate logistic regression analysis (
Table I
Univariate logistic regression analysis of clinical variables and stroke outcomes at 30 days and 90 days.
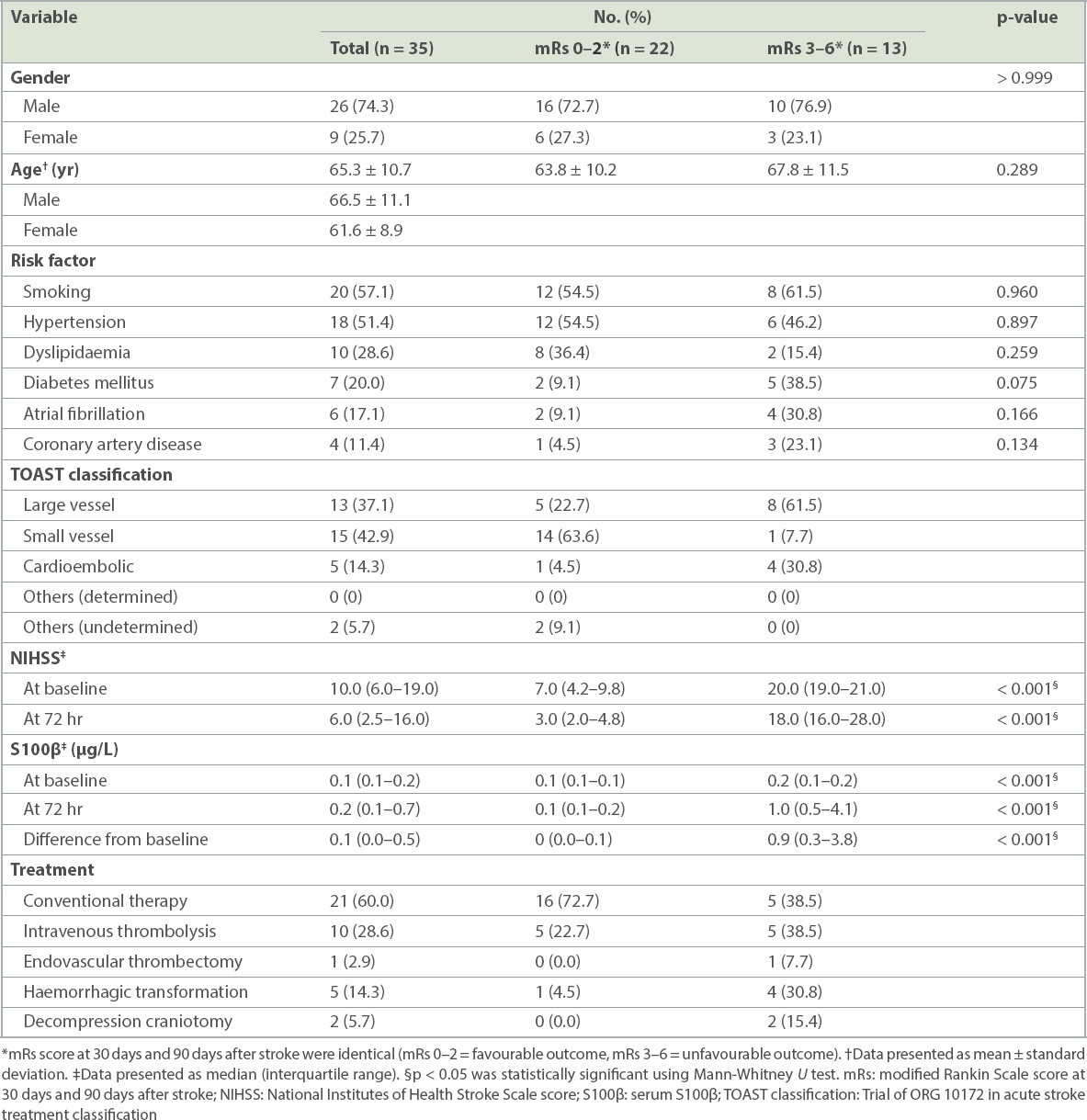
Fig. 1
Charts show the correlation of serum S100β at baseline with (a) NIHSS score at baseline; (b) mRs score at 30 days after stroke; and (c) mRs score at 90 days after stroke. mRs: modified Rankin Scale; NIHSSb: National Institute of Health and Stroke Scale score
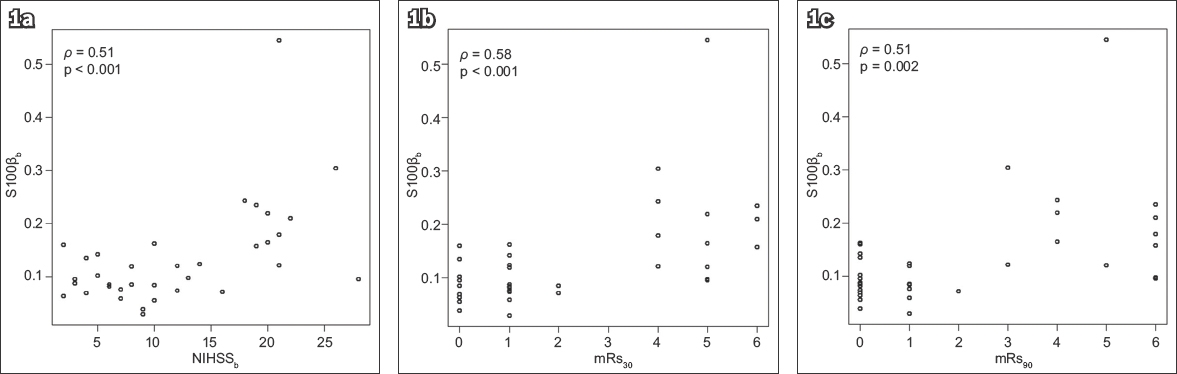
Fig. 2
Charts show correlation of serum S100β at 72 hours after stroke onset with (a) NIHSS score at 72 hours after stroke onset; (b) mRs score at 30 days after stroke; and (c) mRs score at 90 days after stroke. mRs: modified Rankin Scale; NIHSS: National Institutes of Health Stroke Scale score
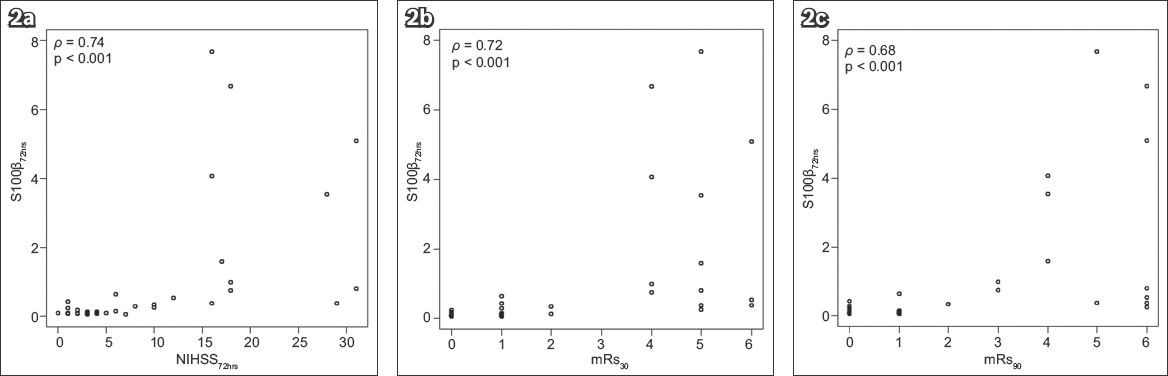
Fig. 3
Charts show correlation of ∆S100β with (a) ∆NIHSS; (b) mRs score at 30 days after stroke; and (c) mRs score at 90 days after stroke. ∆NIHSS: difference between National Institutes of Health Stroke Scale score at baseline and at 72 hours after stroke onset; ∆S100β: difference between serum S100β at baseline and at 72 hours after stroke onset; mRs: modified Rankin Scale
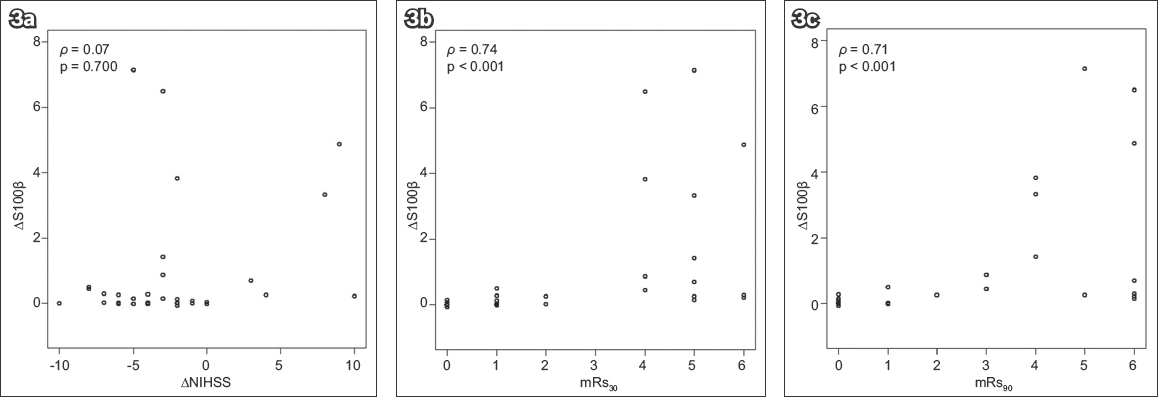
The optimal cut-off points for S100βb, S100β72hrs and ∆S100β for the prediction of unfavourable stroke outcomes and death, as well as sensitivity, specificity, area under the curve, positive predictive value and negative predictive value identified by the ROC curves, are presented in
Table II
Optimal cut-off points for serum S100β at baseline and at 72 hours after stroke onset for predicting unfavourable stroke outcome (mRs > 2) and death (mRs 6) at 30 days and 90 days after stroke.
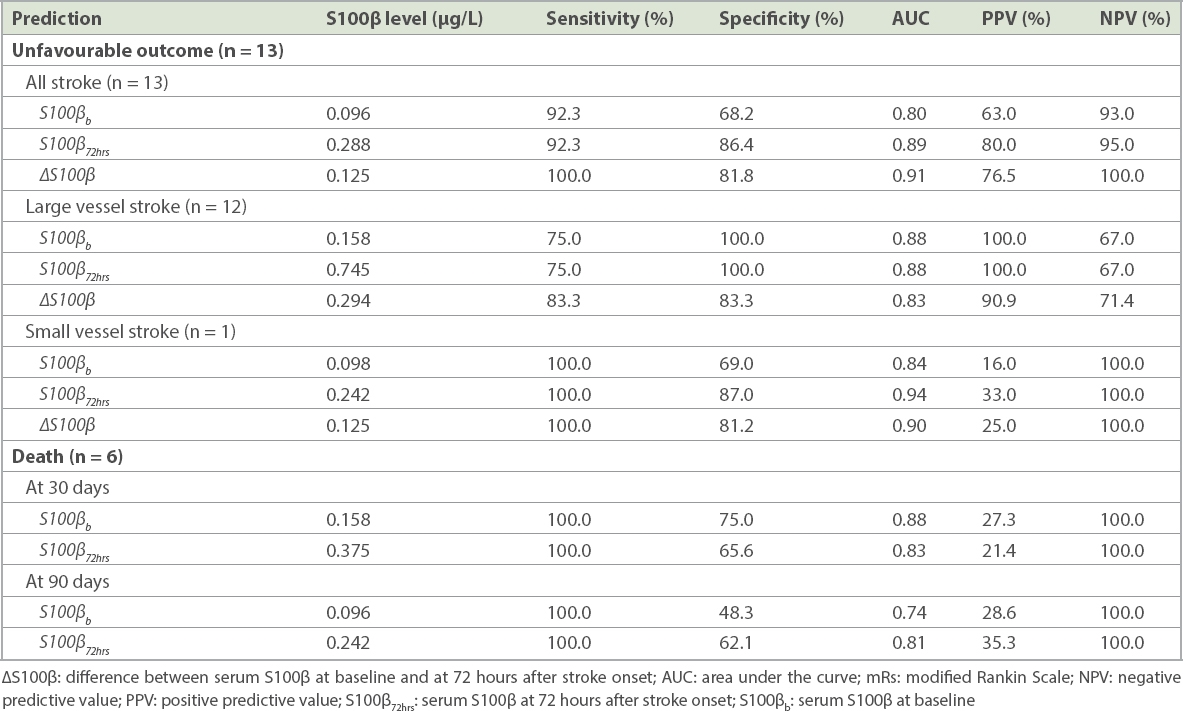
DISCUSSION
Unlike most previous studies that involved large vessel thrombotic and embolic strokes, 17 (48.6%) of 35 patients in our study were classified as SVS. We found that S100βb, S100β72hrs, ∆S100β, NIHSSb and NIHSS72hrs had a significant association with final stroke outcomes upon univariate logistic regression analysis. However, statistical significance was not found following multivariate logistic regression analysis of all NIHSS and S100β values. Therefore, S100βb and S100β72hrs may not be superior to NIHSSb and NIHSS72hrs for predicting the final functional outcome of patients with stroke.
Several widely reported previous clinical studies regarding the usefulness of S100β and other more specific neuronal biomarkers were based on patients with LVS and mostly middle cerebral artery occlusion. Foerch et al(16) reported a correlation between a single S100β measurement and functional outcome and also brain infarction volume seven days after stroke. They also found that the S100β level was not influenced by variables such as age, gender, stroke severity, aetiology, size of lesion and risk factors upon multivariate logistic regression analysis.(16) Furthermore, a single-measurement study of S100β at 48 hours after stroke onset reported that S100β level ≤ 0.37 µg/L could predict an independent functional outcome, with a sensitivity of 87% and specificity of 78% for patients with middle cerebral artery infarction.(16) Another study confirmed that a S100β cut-off level > 0.2 µg/L at 48 hours after stroke in patients with AIS was a strong predictor for unfavourable functional outcome at three months after stroke onset, with a sensitivity of 85% and specificity of 92%. Moreover, a study demonstrated that elevation of S100β as early as six hours after stroke onset was associated with an unfavourable functional outcome.(12) Fassbender et al(8) showed a correlation between elevated serum S100β levels at ten hours, 24 hours and 72 hours after stroke onset with an infarction volume > 5 mm3 and functional outcome. In their study, the elevation of S100β was found to be significantly higher for cortical infarctions than for subcortical and brain stem infarctions at 24 hours and 72 hours after stroke onset.(8)
In our study, the first blood sample for the S100β assay was collected within 12 hours and, significantly, a second sample was collected at 72 hours after stroke onset, which was considered the most optimal time point to assess S100β levels for estimating the extent of cerebral damage after stroke. This time point for the second S100β measurement was selected based on the findings of a previous experimental animal study and a clinical review of the role of S100β in AIS.(17,19) Although the level of S100β in our study was not identical to previous studies, we found a comparable correlation with stroke severity as well as short- and long-term functional outcomes, as evaluated by mRs30 and mRs90, among our patients. Similarly, ∆S100β, in our study, showed a correlation with mRs30 and mRs90.
The cut-off values of S100βb, S100β72hrs and ∆S100β were good predictors of unfavourable outcome for all stroke subtypes in our study. However, as only one patient with SVS who experienced unfavourable outcome was included, our findings vis-à-vis the preciseness and generalisation of the cut-off values for S100β to all patients with stroke may be limited. Additionally, the cut-off values found for S100β72hrs and ∆S100β were notably more appropriate than S100βb in predicting an unfavourable functional outcome. Therefore, similar to previous studies,(8,17,19) we also propose that S100β measurement is appropriate for the prediction of unfavourable stroke outcome among patients with LVS, while its usefulness in patients with SVS remains as yet undetermined. Furthermore, a single measurement at 72 hours, or S100β72hrs, has the highest specificity for predicting an unfavourable outcome of AIS.
Most studies have recognised the clinical usefulness of assessments of S100β levels for estimating the extent of cerebral ischaemic damage that is reflected in infarction volume, monitoring patients’ response to treatment and their subsequent functional outcome, as assessed by mRs.(6,8,12,15,16,18,20) The S100β level can be detected in the serum as early as 6–12 hours after stroke onset and, in most patients, reaches its peak 24 hours or more after stroke.(18) However, some other studies have reported the S100β peak at 48–72 hours after stroke onset,(8,9,12,17) suggesting that there is as yet no consensus over the optimal time points or number of S100β sample assays to be rationally performed for patients with stroke. On the contrary, certain studies have suggested that cerebrospinal fluid might be a more appropriate source for S100β and NSE assessments to predict stroke severity since, with cerebrospinal fluid measurements, there is no interference from other potential extracranial sources of S100β such as adipose tissue, melanocytes, T-lymphocytes, skin or skeletal muscles.(2,11) In view of these earlier findings, physicians should exercise caution when using S100β and NSE measurements for evaluating patients with stroke unless such potential extracranial sources are carefully excluded.
Other biomarkers for the prediction of stroke outcome have also been investigated. Jauch et al, in the National Institute of Neurological Disorders and Stroke Recombinant Tissue Plasminogen Activator Stroke Study, demonstrated a positive association between the peak 24-hour level of myelin basic proteins, NSE, S100β and NIHSS at presentation, as well as a worse outcome at three months after stroke among patients with a higher difference of S100β and myelin basic proteins levels between 24 hours and two hours after initiation of intravenous thrombolysis treatment.(6) Although NSE followed the same kinetic pattern as S100β, it showed no correlation with infarction volume and functional outcomes,(8) suggesting that NSE has no clinical efficacy for the prediction of outcome of AIS.
A difference in the elevation of biomarkers among patients with LVS and SVS has not been clearly elucidated.(2) A study on glial tissue-specific proteins suggested that glial fibrillary acidic protein was more sensitive for estimating brain damage in patients with SVS, whereas S100β was more suitable for patients with LVS.(11)
The present study, which was conducted in Thailand, demonstrated that S100β had a high specificity for predicting unfavourable outcomes of LVS in an Asian population. Differences in ethnicity have no influence on the S100β measurements. Further clinical investigations enrolling a larger sample size of various stroke subtypes, with particular emphasis on patients with SVS, are warranted to confirm the true clinical usefulness of S100β as a broad stroke biomarker. The optimal number of assessments required and the appropriate time points at which to perform these S100β assessments also need to be specified to standardise practices and to gain a better understanding of the diagnostic and prognostic value of S100β measurements among patients with stroke.
ACKNOWLEDGEMENT
The study was supported by the Research Fund of the Faculty of Medicine, Prince of Songkla University, Songkhla, Thailand.