Abstract
Neurogastroenterology and motility disorders of the gastrointestinal (GI) tract encompass a broad spectrum of diseases involving the GI tract and central nervous system. They have varied pathophysiology, clinical presentation and management, and make up a substantial proportion of outpatient clinic visits. Typically, patients experience persistent symptoms referable to the GI tract despite normal endoscopic and radiologic findings. An appropriate evaluation is thus important in the patient’s care. Advances in technology and understanding of the disease pathophysiology have provided better insight into the physiological basis of disease and a more rational approach to patient management. While technological advances serve to explain patients’ persistent symptoms, they should be balanced against the costs of diagnostic tests. This review highlights the GI investigative modalities employed to evaluate patients with persistent GI symptoms in the absence of a structural lesion, with particular emphasis on investigative modalities available locally and the clinical impact of such tools.
INTRODUCTION
GI motility disorders or functional GI disorders
Patients who present with symptoms suggestive of a disturbance of the gastrointestinal (GI) tract often undergo conventional diagnostic investigations, including endoscopy, radiological imaging and biochemical tests, in order to identify the cause of the symptoms. In the absence of any abnormality, the patient’s condition is regarded as ‘functional’ as opposed to ‘organic’ in nature.(1) While GI motility was previously broadly regarded as a disorder of motor function in the GI tract, we are now more aware of the sensory function of the gut and the complexity of brain-gut interactions in contributing to the symptom complex. The terminology used in functional GI disorders can be confusing. ‘Neurogastroenterology’(2) refers to the study of gastrointestinal muscle and nerve, their regulation, as well as their integration with gastrointestinal function, the central nervous system (CNS) and neuroendocrine system.(3) The term ‘motility’ refers to the motor activity of the digestive tract, including the study of swallowing, gastric emptying, small bowel and colonic transit, and anorectal function. ‘Neurogastroenterology and motility disorders’ is a broader term encompassing the organ systems that result in the symptom complex experienced by patients.(4) These include the CNS, which contributes to the sensory and motor control of the GI tract, and the enteric nervous and smooth muscles of the GI tract.(4)
Neurogastroenterology and motility disorders can be broadly divided into two groups – functional GI disorders (FGIDs) and GI motility disorders. In the absence of anatomical and biochemical abnormalities, patients who complain of symptoms related to the GI tract that are characterised by visceral hypersensitivity are regarded as having FGIDs. On the other hand, GI motility disorders are attributed to underlying neuromuscular pathology, and are characterised by a distinct and measurable alteration of motor function; they generally refer to diseases of GI transit and/or sphincteric function. However both groups are often regarded as ‘functional’, as they typically do not manifest any abnormalities on conventional endoscopy and radiological imaging, and hence, the two terminologies are frequently used interchangeably. Both FGIDs and GI motility disorders share many similar symptoms and may manifest impaired GI physiology.(1,5) Alterations in both motility and sensitivity are believed to be important in symptom generation, resulting in overlapping symptoms in both groups.
FGIDs and GI motility disorders represent an emerging field in Asia, and have gradually attracted increased interest in recent years. The growth in interest is in tandem with the rapid development in research and technological advances, especially in the diagnostic armamentarium, leading to a better understanding of the underlying pathophysiology of these conditions. This has resulted in enhanced patient care in this difficult-to-treat patient population. Both FGIDs and GI motility disorders can affect any region of the GI tract and present with symptoms attributed to a disturbance of gut transit or visceral hypersensitivity. Within the oesophagus, motility disorders include achalasia, scleroderma, hypertensive lower oesophageal sphincter, gastroesophageal reflux disease (GERD) and diffuse oesophageal spasm. Functional dysphagia, functional heartburn and functional chest pain are the commonly encountered oesophageal FGIDs. Gastroparesis is the most frequently encountered motility disorder of the stomach, while functional dyspepsia (FD) is the cardinal FGID arising from the stomach. In the colon and rectum, colonic inertia and Hirschsprung’s disease are organic motility disorders, whereas irritable bowel syndrome (IBS) is considered an FGID.
Motility of the GI tract includes the phenomena of contractile activity, tone, compliance, flow and transit. Sensitivity of the GI tract is intimately related to motility. A range of techniques, both invasive and noninvasive, can be applied to evaluate enteric sensorimotor function in health and disease. Despite the clear pathophysiological distinction between GI motility disorders and FGIDs, symptoms generated by disturbances of gut transit (motility disorders) or visceral sensation (FGIDs) often overlap; hence, it may be extremely difficult to distinguish between the two groups. Patients often suffer from severe GI symptoms, which may mimic structural lesions and lead to significant impairment in the quality of life.(12) Ultimately, diagnostic tests performed for functional GI evaluation are useful in allowing the clinician to evaluate the patient’s symptoms and facilitate a rational approach to patient management.
Yield of functional GI studies
Endoscopy and radiological imaging are first-line investigations. If these investigations do not yield any abnormalities, the performance of more diagnostic tests allows the clinician to further characterise symptoms. Performance characteristics of an ideal clinical investigative modality include: (a) it yields a high diagnostic specificity (i.e. the results of the diagnostic test lead to a specific diagnosis); (b) it provides an explanation for the cause of the patients’ symptoms; (c) it determines the choice of therapy; and (d) it predicts a response to therapy.(13) In this review, we highlight the investigative modalities employed in the evaluation of patients with persistent GI symptoms despite negative endoscopic and imaging findings, with particular emphasis on facilities that are available locally and the clinical impact of such investigative tools.
EVALUATION OF OESOPHAGEAL FUNCTION
Disorders of the oesophagus are mainly due to alterations in neuromuscular function. Significant advances have been made in the understanding of the complex pathophysiology of oesophageal disorders. This is fuelled by the development of various diagnostic modalities that are readily available in most institutions in Singapore. Oesophageal manometry is useful in the diagnosis and classification of oesophageal motility disorders.(14) In the early days, oesophageal manometry was performed with the conventional water-perfused catheter system with 4–8 sensors. Pioneering work by Clouse and Staiano(15) led to the use of pressure topography to analyse and view manometric data from standard line plots to provide information about oesophageal peristalsis, which was not appreciated from conventional line tracings (
Fig. 1
(a) High-resolution manometry (HRM) from line plots to spatial temporal plots.(15) The x-axis represents time, y-axis represents the position along the catheter, and z-axis represents pressure recordings. (b) The concept of HRM. Closely spaced recording sensors on an oesophageal motility catheter generate multiple recordings throughout the oesophagus. Dashed arrows point to pressure recordings from individual sensors. Computer software fills in best fit data between the recording sensors spaced 1-cm apart, and colour codes amplitude levels. Finally, the image is smoothed out electronically and displayed as a topographic contour plot, with ‘D’ representing the peristaltic sequence when viewed from above. The contour plots are termed Clouse plots in honour of Ray Clouse, who developed HRM (reproduced with permission from Wiley © Gyawali CP. Neurogastroenterol Motil 2012; 24 (Suppl 1):2-4).
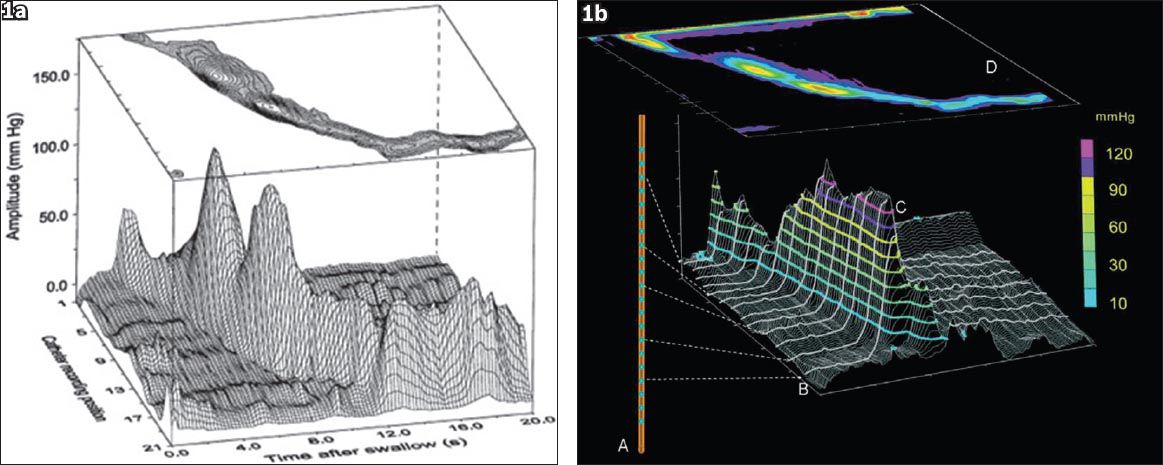
Fig. 2
Distinguishing conventional manometry from high-resolution manometry (HRM) with oesophageal pressure topography. (a) The catheter in this example has 30 pressure sensors spaced 1-cm apart along the length of the catheter. (b) The data output for HRM would be in the form of a line-tracing format providing a measure of contractile strength on a vertical axis. The red lines depict what the conventional manometry spacing would be if the sensors were spaced at 5-cm intervals. (c) Clouse plots: The line tracing format pressure data is converted into pressure topography by converting the pressure signal into a colour. Using an isobaric contour tool to isolate all the pressure above 30 mmHg, the anatomic landmarks can be identified: the upper oesophageal sphincter (UES); lower oesophageal sphincter (LES) and oesophago-gastric junction (EGJ) high-pressure zones. There are three distinct segments of contraction along the contractile wave – front separated by three distinct pressure troughs highlighted by P (proximal or transition zone), M (middle), and D (distal). The start of the swallow is highlighted by the dotted yellow line at the start of UES relaxation, and this is an important temporal landmark in oesophageal pressure topography (EPT) analysis. The contractile deceleration point (CDP) is a time point along the contractile wavefront and signifies a transition from the oesophageal body to LES function. The distal latency is calculated by measuring the time between the start of the UES relaxation and the CDP. Contractile front velocity (CFV) assesses propagation, and is similar to the standard peristaltic velocity, with the caveat that the measurement is confined to the oesophageal body domain between the proximal trough and the CDP. Contractile vigour is calculated differently using EPT: the distal contractile integral (DCI) quantifies the contractile activity within the space time box highlighted by the white dotted box. It calculates the pressure activity above 20 mmHg to exclude artifact and intrabolus pressure, and is presented using a unit of mmHg-cm-s (reproduced with permission from Wiley © Gyawali CP, et al. Neurogastroenterol Motil 2013; 25:99-133).
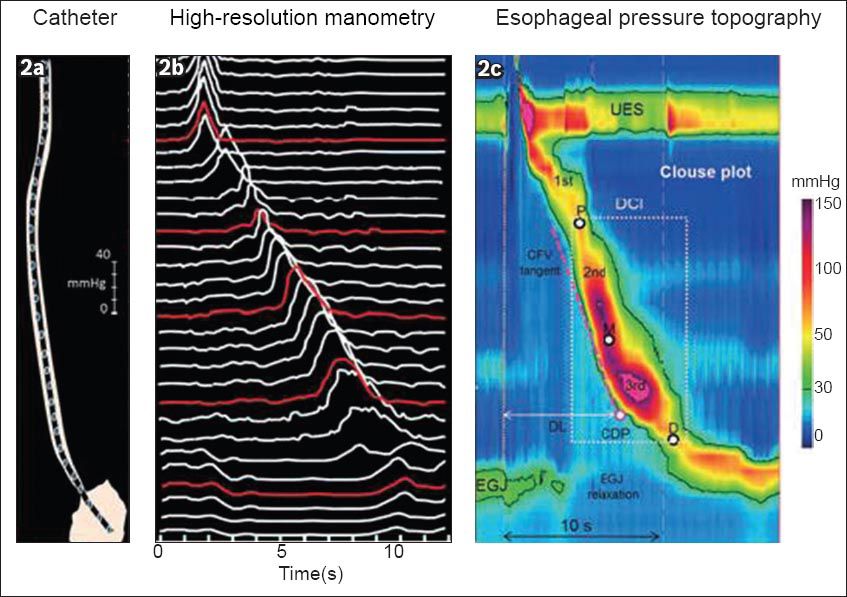
Fig. 3
Example of a HRM tracing illustrating normal peristalsis.
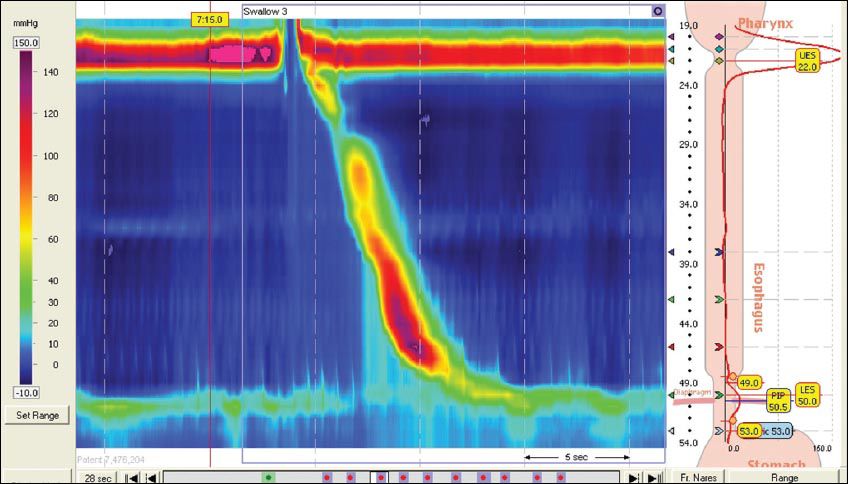
Acid reflux (AR) and non-acid reflux (NAR)-related events in the oesophagus continue to be an area of intense research. While GERD used to be easily treated with proton pump inhibitors (PPIs), gastroenterologists are now encountering an increasing number of patients who have a normal endoscopy but continue to have reflux-like symptoms, including heartburn and regurgitation, and who do not respond well to PPIs. In this setting of PPI-refractory symptoms, functional oesophageal tests are useful to guide further management. Unlike the conventional 24-hour nasopharyngeal pH catheter system (
Fig. 4
Photograph shows a conventional 24-hour pH catheter system that measures only acid reflux.
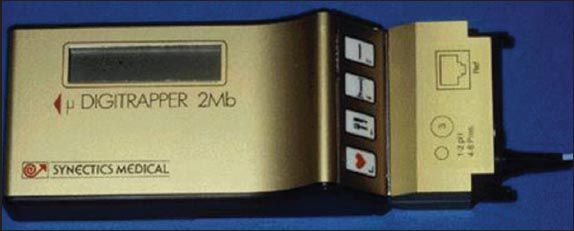
Fig. 5
The wireless pH (Bravo™) capsule (Medtronic Inc, Minneapolis, MN, USA) measures 6 mm × 5.5 mm × 25 mm. The capsule attaches to the oesophageal wall and transmits pH data via radiofrequency signal to a small receiver attached to the patient’s belt.
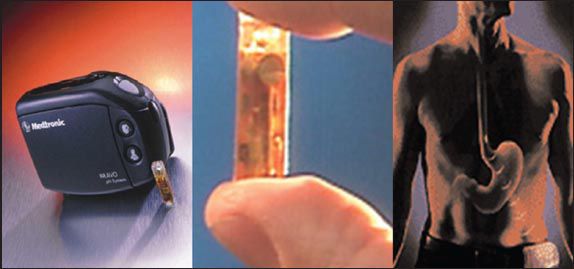
Fig. 6
(a) Diagram illustrates the principles of intraluminal impedance-pH monitoring. In this technique, resistance to an alternating current is measured at multiple sites along the oesophagus. This is performed with a thin catheter mounted with ring electrodes. MII-pH detects the presence of a bolus by means of changes in the conduction between electrodes. Bolus contents that are liquid and mixed have a low impedance, and gas contents have a higher impedance. By using several pairs of electrodes, the detection of bolus movement is possible. The reflux can further be categorised by the pH electrode into acid (pH≤ 4) or non acid (pH > 4) (reproduced with permission from Elsevier © Savarino V. Dig Liver Dis 2004; 36:565-9). (b) Combined multichannel intraluminal impedance and pH (MII-pH) monitoring. Example of an acid reflux episode. Figure illustrates impedance measuring channels centred at 3, 5, 7, 9, 15 and 17 cm above the lower esophageal sphincter (LES). MII-pH identifies reflux episodes as rapid decline in intraluminal impedance progressing over time from distal to proximal (arrow). Information from the pH channel is used to distinguish between acid (nadir pH < 4) and non-acid (nadir pH > 4) reflux episodes. (c) Example of a non-acid reflux episode.
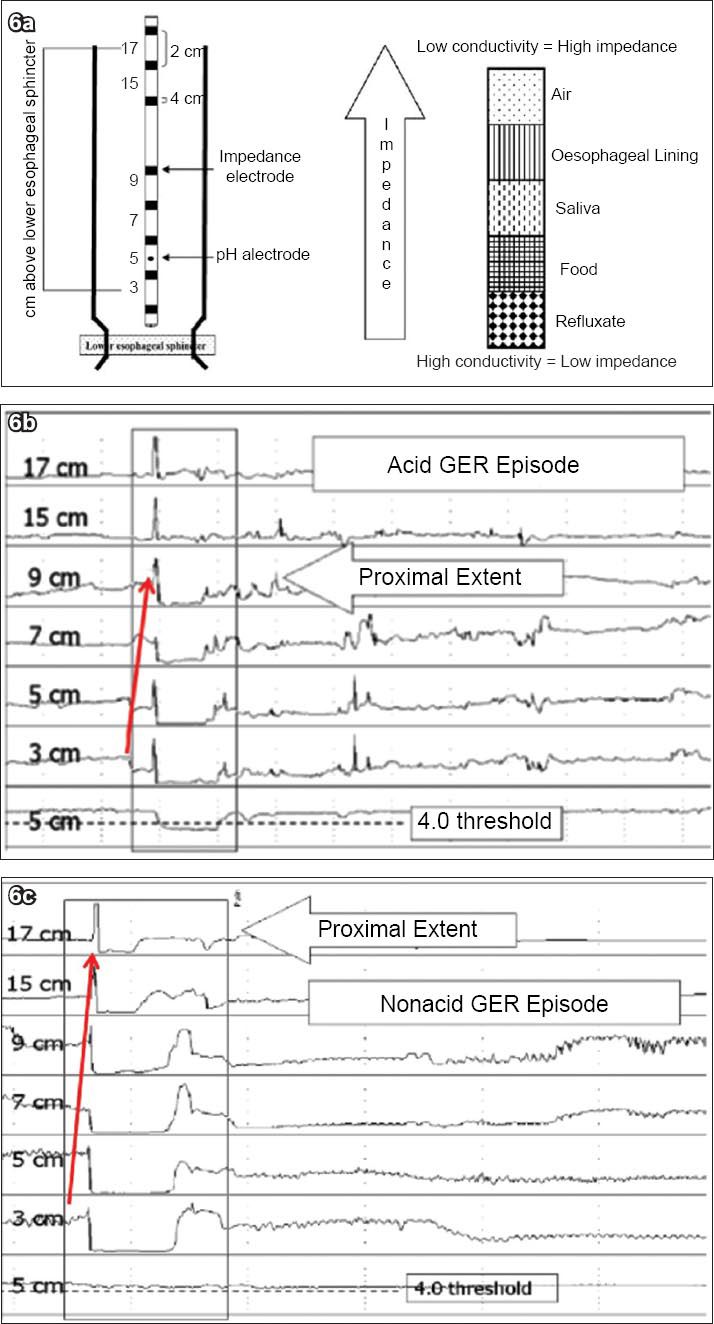
EVALUATION OF GASTRIC FUNCTION
Dyspepsia is a symptom complex that is defined as persistent or recurrent abdominal pain or discomfort centred in the upper abdomen. If the symptoms persist for more than three months without an anatomical or a biochemical abnormality, this would fulfil the definition of FD.(7) Many surveys have evaluated the prevalence of dyspepsia in the community, with rates varying depending on the definitions used. The prevalence rate has been estimated to be approximately 15%.(28) Several pathophysiological mechanisms have been proposed to underlie symptom generation in FD. Gastric symptoms can be attributed to abnormal gastric emptying, impaired gastric accommodation, heightened gastric sensation, or alterations in gastric myoelectrical function and contractility.(29) Investigations in gastric motility are generally pursued only after exclusion of an organic cause and failure to respond to PPIs and prokinetic agents.
Gastric emptying
Measurement of the rate of gastric emptying is a test that is most frequently used to access gastric function. The gold standard for evaluation of gastric emptying is the gastric scintigraphic study, for which important consensus guidelines are available.(30) The percentage of gastric emptying is measured using scintigraphic images at intervals of up to 4 hours after ingestion of a radioisotope-labelled meal. The gastric residue at 4 hours has been shown to be the most accurate parameter for the detection of delayed gastric emptying, with a sensitivity and specificity of 100% and 79%, respectively.(30) However, the disadvantages of this method are the need for the use of radioactive labels, high costs and the lack of standardisation of meals across centres. To circumvent exposure to radiation, the 13C-octanoate-breath test, an alternative method that has shown good correlation with the conventional scintigraphic study, can be used.(31-33) This test employs a standardised meal labelled with 13C-octanoic acid. Following gastric emptying, the 13C substrate is absorbed and rapidly metabolised in the liver, generating 13CO2. By measuring 13CO2 in expired breath samples taken at regular intervals, it is possible to calculate a gastric emptying curve. More recently, the wireless SmartPill® GI Monitoring System (SmartPill Corporation, Buffalo, NY, USA) allows the evaluation of regional and whole gut transit times. Using the wireless SmartPill, gastric emptying is measured as the time interval between ingestion of the capsule and an abrupt increase in pH > 3 pH units from gastric to baseline.
However, the relationship between gastric emptying and symptom pattern is as yet controversial.(34,35) Studies have not shown a correlation between the severity of delayed emptying and the response to prokinetic therapy. More recently, rapid gastric emptying has been implicated in FD patients.(36,37) The mechanism for this phenomenon has been attributed to enhanced antral contractility, decreased duodenal feedback inhibition and impaired accommodation.(38) Hence, FD symptoms may be attributed to either delayed or rapid gastric emptying. In routine clinical practice, patients who have symptoms suggestive of dysmotility or gastroparesis are often treated empirically with prokinetic drugs, regardless of the results of the gastric emptying test. Dietary measures and somatostatin analogues are, however, rarely used in cases suggestive of rapid gastric emptying, such as in dumping syndromes. Therefore, gastric emptying tests, although not routinely recommended, are useful for patients who continue to experience persistent symptoms despite having received empirical treatment, as this allows the gastroenterologist to further characterise the symptoms and employ more invasive treatment modalities, such as more intense prokinetic therapy or experimental treatment in the appropriate research setting.
Gastric accommodation
The prevalence of impaired gastric accommodation in patients evaluated for FD is approximately 40%.(39,40) The conventional gold standard for the evaluation of impaired gastric accommodation involves the use of a gastric barostat. This involves the insertion of a double lumen polyvinyl tube with an adherent plastic bag through the mouth into the stomach. The balloon is unfolded by inflation of 500 ml of air and positioned in the proximal stomach by gently withdrawing the tube until slight resistance is felt. The tube is connected to the barostat, allowing for the measurement of changes in gastric tone and sensitivity. In this way, the gastric accommodation response to various interventions can be evaluated. However, impaired gastric accommodation is not specific for dyspepsia, as it may be seen in various conditions, such as FD, diabetic gastropathy, rumination syndromes and post-Nissen fundoplication, as well as in patients with anxiety. In addition, the invasive nature of the gastric barostat and the discomfort associated with this investigative modality have limited the performance of this test only to tertiary referral centres within a research setting.
Gastric volume imaging techniques using ultrasonography,(41,42) as well as single-photon emission computed tomography(43) imaging have enabled the quantitative assessment of changes in proximal stomach volume after a meal, with the assumption that any changes in proximal stomach volume after a meal reflect meal-induced gastric accommodation. Gastric magnetic resonance (MR) imaging has been used to measure gastric emptying and to evaluate gastric motor function noninvasively without exposure to radiation.(44) However, the high costs of these modalities and their limited availability have largely restricted their use to research settings.
Electrogastrography
The electric potentials or slow waves that underlie motor activity in the stomach can be measured with cutaneous electrodes. Electrogastrography (EGG) provides information on the frequency and regularity of the gastric pacemaker activity.(45) Special methodologies are required to acquire, amplify and filter the signals. Gastric dysrhythmias are recognised when the electrical frequency is less than (bradygastria) or more than (tachygastria) 3 cycles per minute. Although the EGG is a noninvasive method of measuring gastric electrical activity, there has been limited correlation between EGG and gastric emptying/contractility. In addition, EGG abnormalities can be induced by non-GI causes.(46) Hence, the role of EGG has also primarily been restricted to research settings.
Evaluation of small bowel function
The time interval between ingestion of a non-absorbable carbohydrate (lactulose) and its arrival in the caecum is the orocaecal transit time, which can be measured using hydrogen excreted in the breath.(47) Although initial breath tests relied on the measurement of labelled CO2,(48) difficulties encountered when correcting for endogenous CO2 had affected the accuracy of these tests.(49) In addition, the process of conjugating substrates with labelled carbon is costly. For these reasons, CO2 had breath testing has largely been abandoned in clinical practice.(49)
The lactulose hydrogen breath test serves as a surrogate marker of orocaecal transit times. In this test, oral lactulose passes undigested through the small bowel to the colon, where it undergoes fermentation by colonic bacterial to form short-chain fatty acids, including hydrogen and/or methane gas, which is absorbed systemically and excreted in the breath. Most centres use lactulose 10 g and a 2- to 4-hour breath sampling period. A positive test is defined as: (a) fasting hydrogen levels > 20 ppm; (b) the presence of a double peak, where the first peak represents lactulose metabolism by small intestinal bacteria and the second peak corresponds to lactulose reaching the caecum; and (c) early rise in breath hydrogen concentration > 20 ppm.(50,51) Unfortunately, the lactulose hydrogen breath test is associated with significant limitations. The classic double peak is rarely encountered in clinical practice. In most instances, breath tests are unable to distinguish small bowel from colonic metabolism of substrates.(50) Rather, a single peak in breath hydrogen is found, and this may result from fermentation occurring either in the small bowel (in patients with small intestinal bacterial overgrowth) or in patients with IBS-diarrhoea who have an accelerated ileocaecal transit. The sensitivity and specificity of the lactulose breath test are 68% and 44%, respectively,(52) rendering this test inadequate for routine clinical practice as compared to small bowel culture. Other compounds that have been used for the evaluation of small intestinal bacterial overgrowth, including D-xylose and 13C-xylose, have yielded conflicting results. In view of the significant limitations associated with breath tests, they are not recommended for routine clinical use.
Manometry studies of the small bowel (
Fig. 7
(a) Antro-pyloro-duodenal jejunal manometry. Example of a normal migrating motor complex (MMC) pattern in the fasting state (night) of a normal subject. The upper 3 recordings are from the antrum-pylorus, the lower 3 recordings are from the duodenum-jejunum. (b) Example of a normal MMC pattern in the fasting state of a patient with enteric neuropathy. In the latter, abnormal neurogenic motor activity is seen with disruption of the normal MMC pattern with abnormal activity fronts, bursts of uncoordinated contractions, and sustained uncoordinated contractions.
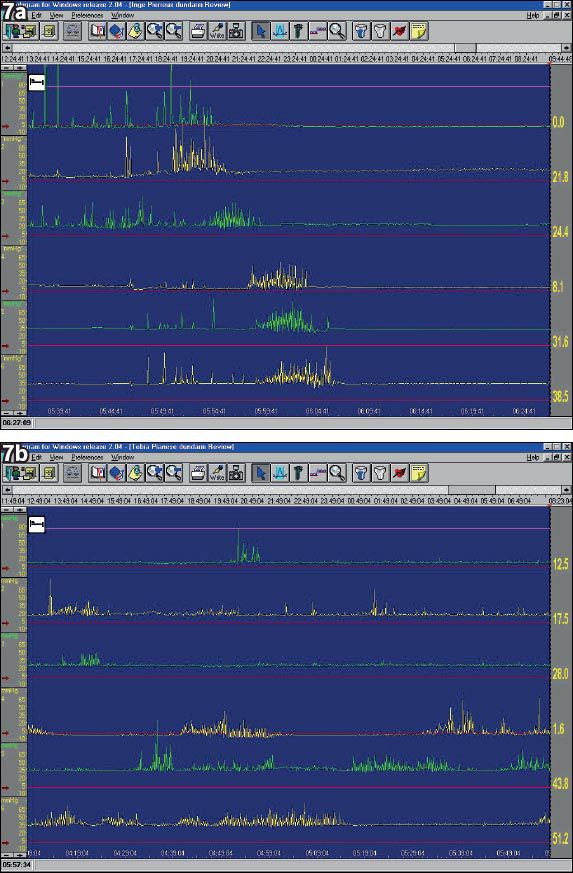
Evaluation of colonic and anorectal function
Gastroenterologists frequently encounter patients with pelvic floor disorders, which can affect 10%–15% of the population.(54) Disorders of colonic motility typically present with constipation and diarrhoea. In the evaluation of patients with constipation who have not responded to a high-fibre diet or over-the-counter laxatives and after the exclusion of organic causes, colonic transit tests using radio-opaque markers (ROM) can easily be performed. The ROM test is a simple and non-invasive method to differentiate normal transit from slow transit constipation. Although this test measures whole gut transit time (WGTT), it is also used as an estimate of colonic transit time, as most of the WGTT reflects the passage through the colon.(55) Different protocols have been described, including the Hinton(56) and Metcalf(57) techniques. In the former technique,(56) ingestion of a known number of pellets (either 20 or 24) is followed by single abdominal radiography after 120 hours (Day 6). Slow transit is defined by the presence of ≥ 20% of pellets in the gut.
Anorectal manometry (ARM) provides valuable information on the underlying pathophysiology of constipation in patients who have unexplained constipation that did not respond to laxatives, and dietary and lifestyle measures. It is also useful in patients with faecal incontinence after established diagnostic tests (including endoscopy and stool studies) are unyielding.(58) ARM study involves the insertion of a catheter with pressure sensors and a balloon on the catheter tip. Resting and squeeze pressures and the rectoanal gradient (the difference between rectal and anal pressures) are measured. This is followed by asking the patient to bear down and attempt to defaecate. The rectal balloon is subsequently inflated with 30–50 ml of air to evaluate for the rectoanal inhibitory reflex (
Fig. 8
(a) High-resolution anorectal manometry of a normal bearing-down attempt shows an increase in rectal pressure and a decrease in pressure of the anal sphincter. (b) Anorectal manometry of a patient with obstructed defaecation (pelvic floor dyysnergia) shows paradoxical contraction of the anal sphincter during the bearing-down manoeuvre.
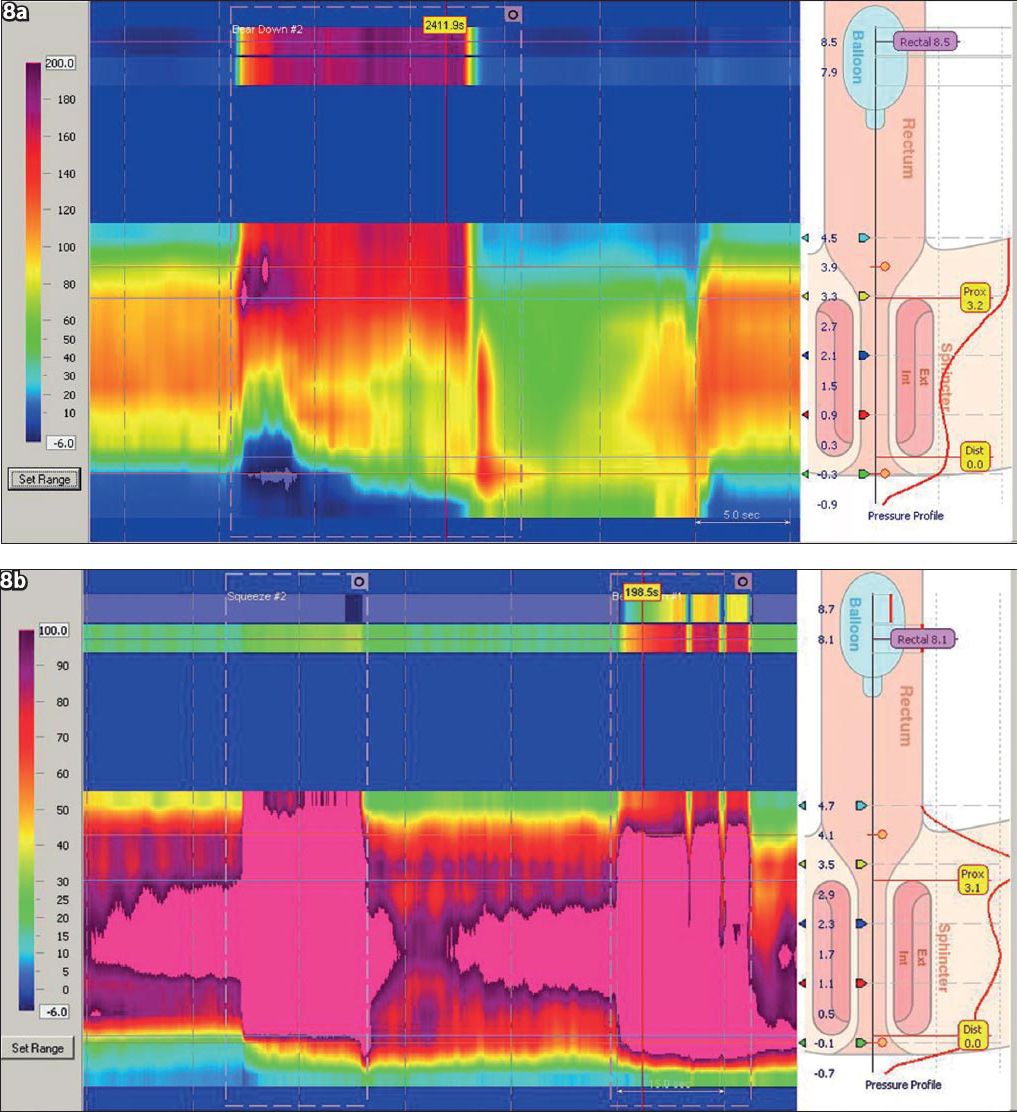
Patients who have pelvic floor dyssynergia (
Despite these technological advances, the inherent limitations of ARM deserve mention. There is considerable overlap in the rectoanal gradient between asymptomatic subjects and patients with pelvic floor dyssnergia. The lack of privacy and the act of artificially simulating rectal evacuation in the left lateral supine position in a laboratory setting may contribute to overlapping results between asymptomatic controls and patients. In addition, there is no single criterion used to diagnose defaecation disorders (DDs). In a recent meta-analysis of 7,591 patients with constipation, the prevalence of findings that suggested abnormal defaecation was varied – from 14.9% for absent opening of the anorectal angle on defaecography to 47.7% for a dyssynergic pattern on anorectal manometry and 52.9% on ultrasonography.(62) These findings highlight the considerable heterogeneity of DDs and their associated challenges. Thus, test results must be interpreted in the overall clinical context, taking into account local facilities and costs. Ultimately, the impact of diagnosing DDs is that it enables the clinician to tailor the appropriate treatment. Treatments such as biofeedback and pelvic floor retraining in patients with DDs have been well established.(11)
Defaecography provides valuable information on the anorectum.(63) In this procedure, barium is injected into the rectum, and the patient is asked to expel the volume of barium. Information gained from this modality includes the identification of an enterocoele, rectoanal gradient and pelvic floor descent. To avoid radiation exposure, MR defaecography(64) can be performed; however, this test is not available in Singapore.
Novel diagnostics
Technological advances now enable us to evaluate patients in an ambulatory setting with minimal interference of daily activities. The wireless Bravo pH capsule measures oesophageal acid exposure over a prolonged monitoring period. In recent years, the wireless SmartPill system concurrently measures pressure, pH and temperature, and allows for the evaluation of gastric emptying time, small bowel transit time, colonic transit time and WGTT. This system consists of a wireless motility capsule, data receiver, docking station, computer and data analysis software. The SmartPill capsule (26.8 mm × 11.7 mm), which contains sensors for pH, temperature and pressure, wirelessly transmits this information at 434 MHz using radiofrequency technology. The SmartPill is approved by the US Food and Drug Administration (FDA) for the measurement of gastric emptying in patients with suspected gastroparesis.(65) It has shown performance characteristics that are comparable to the gastric emptying test(66) and the ROM test(56,57) in the evaluation of WGTT and colonic transit time. More recently, the SmartPill has been compared with conventional ROM in assessing patients with slow transit constipation based on symptoms. Good correlation was observed between the wireless motility capsule and the ROM test (r = 0.718, p = 0.0001).(67) The SmartPill has also been directly compared with conventional antroduodenal manometry (ADM) in assessing gastric and small bowel MMCs. In Brun et al’s study,(68) healthy subjects underwent insertion of the ADM catheter, and following ingestion of a standard meal, they then swallowed the SmartPill. Using the 10th amplitude percentile contractions as pressure thresholds, the sensitivity and specificity of high amplitude contractions recorded on the SmartPill, which represents phase III MMCs, were respectively 90% and 72% in the stomach and 74% and 85% in the small bowel, with negative predictive values of 99.9% in both regions. Thus, the authors concluded that the SmartPill has the potential to serve as a screening tool in patients with suspected small bowel dysmotility.(68)
CONCLUSION
Neurogastroenterology and motility has moved from an obscure niche in academic gastroenterology(69) to an area of intense research. These disorders make up a substantial proportion of clinic visits encountered by the gastroenterologist, and their severity ranged from mild to conditions that significantly impact the affected patients.(70) Measurement techniques are constantly evolving with the times, but one must acknowledge that despite the technical advances made, not all the latest developments have translated into daily clinical practice. The clinical relevance of EGG remains unproven, while the use of complex imaging techniques like 3-dimensional ultrasonography and MR imaging are limited to only a few centres in the world. Thus, when evaluating patients with disordered sensorimotor function, the diagnostic modality used is often guided by local expertise and available facilities. Other factors to consider include the diagnostic specificity of the test, the ability to find a cause for the symptoms experienced, and the ability of the test to influence the choice of therapy.(13)
Table III
Summary of functional gastrointestinal (GI) tests available locally.
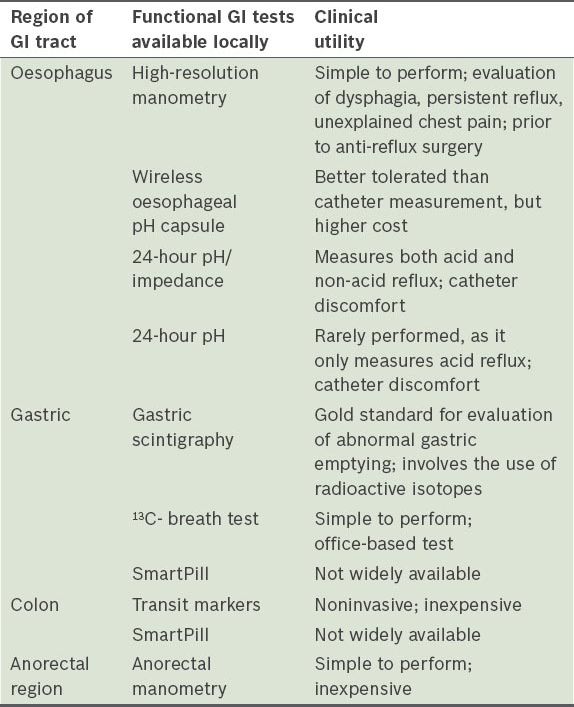
The main limitation of functional GI studies is the lack of diagnostic specificity. Hence, they are best used in the context of providing an explanation for symptoms that persist after exclusion of a structural lesion on endoscopy and radiological imaging. This in turn helps the clinician to tailor the appropriate therapy. In the appropriate clinical setting, the role of functional GI evaluation lies in providing an explanation for the cause of symptoms, which helps to alleviate patients’ concerns, reassuring them that there is no serious underlying organic pathology, as well as guide the gastroenterologist to implement the appropriate therapy. To date, this remains the most important clinical impact of functional GI evaluation.